KIDA LABORATORY
Department of Applied Chemistry, Graduate School of Engineering, Osaka University
大阪大学大学院工学研究科応用化学専攻
分子創成化学講座 木田研究室
Research
- Development and Application of Cyclic Oligosaccharide Derivatives Bearing High Guest Inclusion Ability in Organic Media
- Creation and Application of CD Supramolecular Structures
- Development and Application of Skeleton-Modified CDs
- Creation of Polymer Tubes through One-Dimensional Fusion of Polymer Capsules
- Chiral Photochemistry through the Charge-Transfer Band Excitation
- Development and application of highly luminescent circularly polarized emitting molecules with molecular recognition properties
- Creation and utilization of supramolecular photocatalysts through self-assembly of organic dye molecules
1.Development and Application of Cyclic Oligosaccharide Derivatives Bearing High Guest Inclusion Ability in Organic Media
Cyclodextrins (CDs), which are produced from starch by a enzyme ‘cyclodextrin glucanotransferase’, are a class of cyclic oligosaccharides consisting of several α-(1,4)-linked D-glucopyranose units. CDs composed of 6, 7, and 8 glucosidic units, called α-, β-, and γ-CD, respectively, are commercially available, and have been studied extensively. They have a doughnut-shaped cavity into which a guest molecule of an appropriate size and shape can be included. The ability of CDs to form inclusion complexes with organic molecules has been studied academically, and, in addition, it has found applications in many areas, including the food, cosmetic, and pharmaceutical industries.
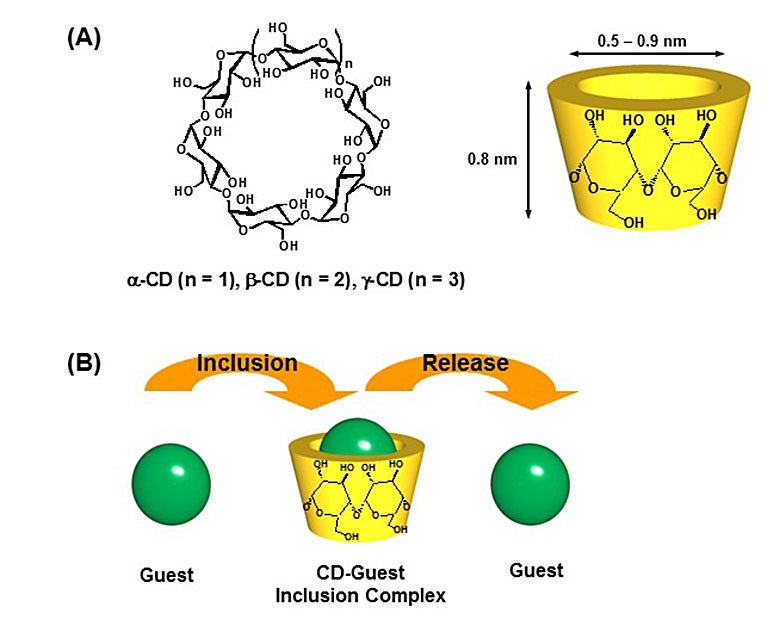
Figure 1. (A) Chemical structures and schematic illustration of of cyclodextrins (CDs). (B) Schematic illustration of the inclusion and release of guest molecules into and from the CD cavity.
CDs can form inclusion complexes with a variety of molecules in aqueous media or in several kinds of polar organic media via the incorporation of the guests into the CD cavities. On the other hand, it has been believed that inclusion complex formation between CDs and guest molecules in nonpolar media would be extremely difficult, because the main driving force for the inclusion of guest molecules within the CD cavity is hydrophobic interactions and/or van der Waals interactions between the guests and the CD cavity, and the enormous amount of nonpolar solvents become a strong competitor for inclusion within the CD cavity. We found that 6-O-modified CDs effectively form inclusion complexes with guest molecules in nonpolar solvents, and demonstrated that these CD hosts functioned as a selective adsorbent for polychlorinated biphenyls (PCBs) contaminated in insulating oils. We also succeeded in the efficient separation and concentration of PCBs from oils by passing the PCBs-contaminated oils through a column loaded with the CD hosts.
More than 500,000 tons of PCB-contaminated oils are still stored in Japan, and thus the development of appropriate treatment methods for these oils is highly required. Our technique with a CD-loaded column can be expected to contribute to a large reduction in the stockpile of PCBs-contaminated insulating oils in Japan. We are now studying to put this technique into practical use.
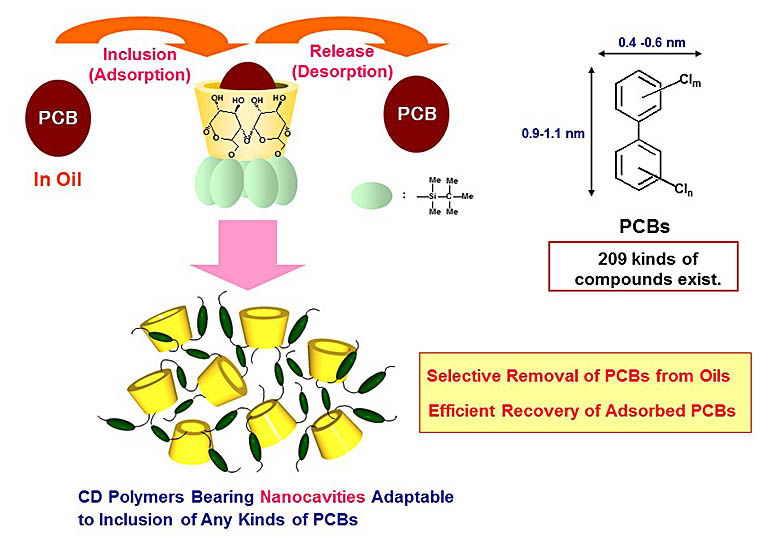
Figure 2. Inclusion complex formation between CD derivatives and PCBs in oils.
- 1) T. Kida et al., Org. Lett. 2009, 11, 5282-5285. 2) T. Kida et al., Org. Lett., 2011, 13, 4570-4573. 3) T. Kida et al., J. Am. Chem. Soc., 2013, 135, 3371−3374. 4) S. Kawano et al., Environ. Sci. Technol., 2014, 48, 8094-8100. 5) C. Kogame-Asahara et al., ChemPlusChem, 2018, 83, 868-873. 6) J. M. Kalaw et al., ChemPlusChem, 2020, 85, 1928-1933.
2.Creation and Application of CD Dimers with Multiple Linkers
A great deal of effort has been devoted to the development of cyclodextrin (CD) dimers to further exploit the functionality of CD and to broaden the range of guest molecules that can be incorporated. In particular, various CD dimers with a single covalent linker have been developed, which can stably incorporate guest molecules larger than the CD monomer cavity. On the other hand, there are very few reports on multilinker-type CD dimers, in which two CD molecules are linked by multiple covalent linkers, due to the difficulty of synthesis. We have succeeded in easily synthesizing a novel β-CD dimer in which two molecules of 6-O–tert-butyldimethylsilylated β-CD (TBDMS-β-CD) are connected by seven m-xylylene linkers (Figure 3). By changing the reagent used to deprotect the TBDMS groups from this β-CD dimer, we succeeded in creating two different types of β-CD dimers: a β-CD dimer in which all TBDMS groups are removed, and a ‘Janus-type TBDMS-β-CD dimer’ in which TBDMS groups on only one of the two CD rings are selectively removed. These β-CD dimers were found to exhibit selective inclusion ability for cis-type long-chain fatty acid esters over trans-type and saturated long-chain fatty acid esters. In particular, the Janus-type TBDMS-β-CD dimer exhibited a very high association constant of the order of 105 for methyl docosahexaenoate (DHA). By designing and synthesizing CD dimers by appropriately selecting the type of CD, the structure and number of linkers and terminal substituent groups according to the structure of the target guest molecule, ultra-precise molecular recognition, which could not be achieved with conventional host molecules, is expected.
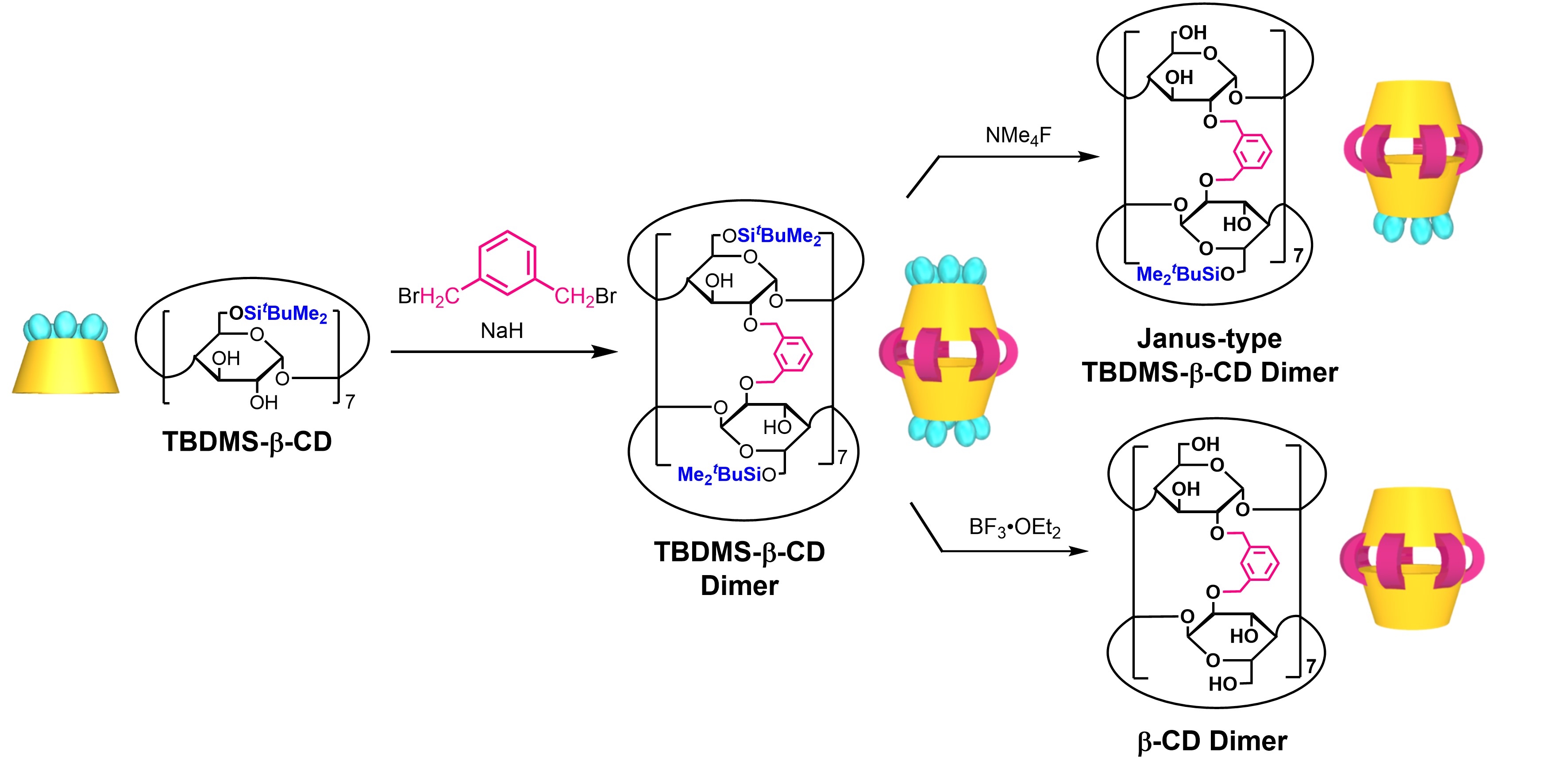
Figure 3. Synthesis of CD dimers with multiple linkers.
- 1) S. Ito et al., Tetrahedron Lett. 2016, 57, 5243-5245. 2) C. Kogame-Asahara et al., Chem. Commun. 2020, 56, 1353-1356. 3) C. Kogame-Asahara et al., ACS Omega, 2021, 6, 3227-3231.
3.Creation and Application of CD Supramolecular Structures
Cyclodextrins (CDs) are known to adopt three assembly modes in crystals: cage, channel and layered. Of these, the channel-type assembly, in which CD molecules are aligned via hydrogen bonds between each hydroxyl groups to form a cylindrical structure, has attracted much attention because it can be used as a molecular container or drug carrier. We have successfully prepared various morphologies of nano- and microstructures consisting of channel-type assemblies of CD by dropping aqueous or 1,1,1,3,3,3-hexafluoro-2-propanol (HFIP) solutions of CD into lower alcohols (poor solvent) (Figure 4). For example, by dropping an aqueous g-CD solution into 2-propanol, microcubes consisting of channel-type assemblies of γ-CD were obtained as precipitates. The microcubes gelated oils and organic solvents at room temperature, and their channel-like nanocavities enabled the incorporation of guest molecules in oil, which was difficult with CD monomers. The size of the γ-CD microcubes can be controlled by the fabrication conditions, and when inorganic anions are incorporated into the γ-CD cavities, the morphology of the resulting supramolecular structure changes to microrods. On the other hand, by dropping a HFIP solution of α-CD into 1-propanol or 2-propanol at room temperature, wire-like or hexagonal plate-like structures consisting of channel-type assemblies of α-CD were formed, respectively. An interesting phenomenon was also discovered: when the hexagonal nanorods obtained by mixing a HFIP solution of α-CD with 2-pentanol were left under high humidity, their morphology changed into left-handed helical microrods. The insertion of water molecules between the upper and lower α-CD molecules arranged in a channel manner is believed to be the key to inducing the helical structure. In this way, CD-CD and host-guest interactions were used to create various forms of supramolecular structures. α-, β-, and γ
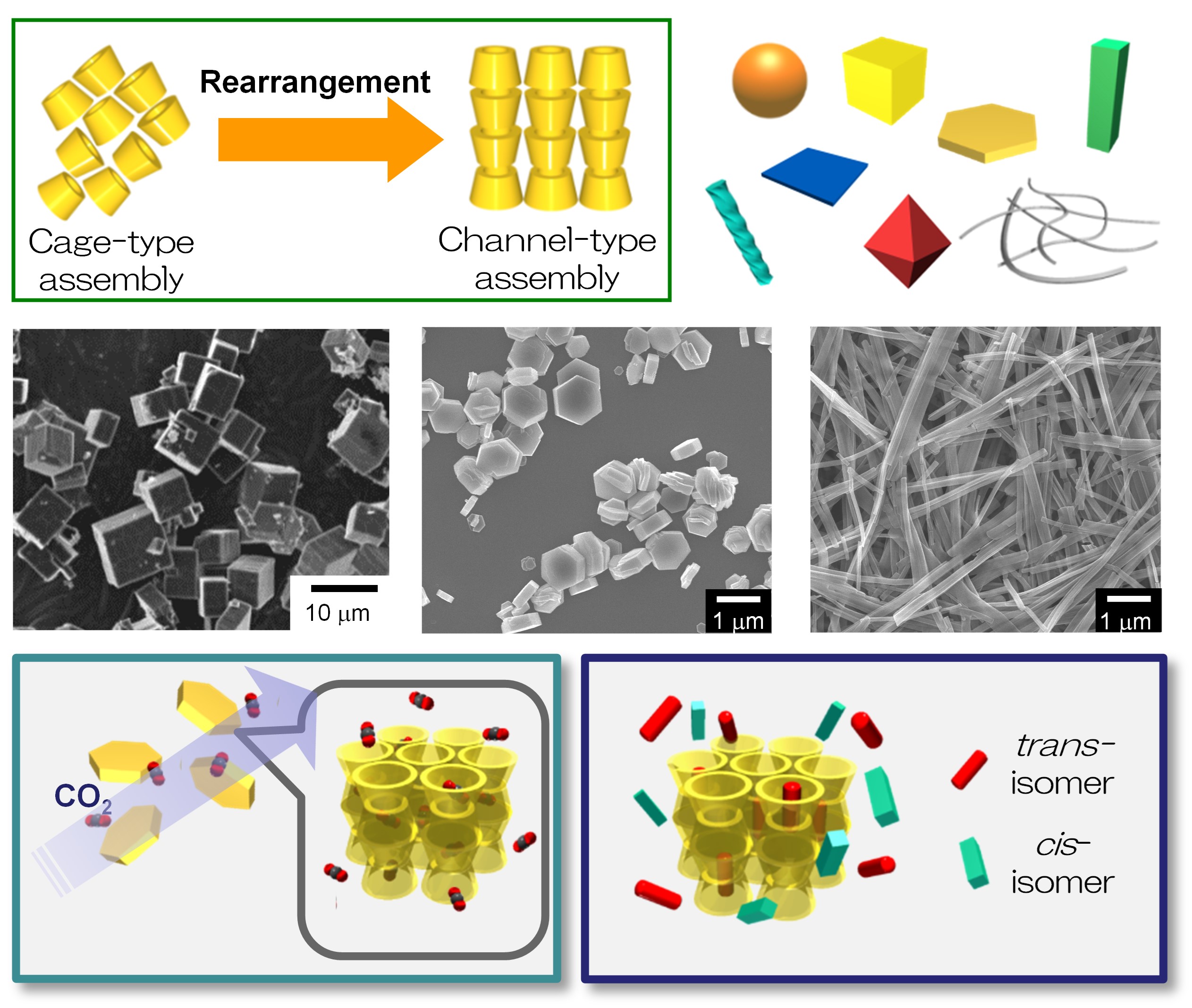
Figure 4. Preparation of CD supramolecular structures with various molphologies.
- 1) T. Kida et al., Anal. Chem. 2008, 80, 317-320. 2) T. Kida et al., Chem. Commun. 2009, 3889-3891. 3) Y. Marui et al., Chem. Mater., 2010, 22, 282-284. 4) Y. Marui et al., Langmuir 2010, 26, 11441-11445. 5) T. Kida et al., Chem. Lett. 2010, 39, 1206-1208. 6) T. Kida et al., Chem. Commun. 2014, 50, 14245-14248. 7) H. Shigemitsu et al., Polym. J. 2018, 50, 541(Review). 8) T. Kida et al., Chem. Commun. 2020, 56, 7581-7584. 9) J. M. Kalaw et al., Langmuir 2022, 38, 5149-5155. 10) J. M. Kalaw et al., Langmuir 2022, 38, 8407-8415. 11) T. Kida et al., RSC Adv. 2023, 13, 34366-34370.
4.Development and Application of Skeleton-Modified CDs
Since it has been believed that flexible design of CD hosts to fit the shape and size of the desired guest molecules would be difficult, the types of guest molecules applied for conventional CD hosts are relatively limited. In order to overcome this drawback and to extend the utility of CDs, we designed ‘spacer-inserted CDs’ whose cavity size and shape are freely controllable, and succeeded in synthesizing them in two steps starting from perrmethylated CDs. These spacer-inserted CDs were found to show unique inclusion ability. ‘Bond-converted CDs’ bearing a β-1,4-glucosidic bond were also synthesized from permethylated CDs. The bond-converted CD derived from perrmethylated α-CD formed an inclusion complex with not only conventional polymeric guests such as polytetrahydrofuran, poly(ε-caprolactone), and poly(propylene glycol) but also with poly(acrylic acid) to generate novel pseudopolyrotaxanes.
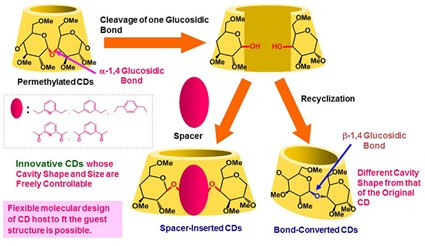
Figure 5. Synthesis of skeleton-modified CDs that are designed to fit the shape and size of guest molecules.
- 1) T. Kida et al., Chem. Commun. 2002, 1596-1597. 2) T. Kida et al., Chem. Commun. 2003, 3020-3021. 3) A. Kikuzawa et al., J. Org. Chem. 2005, 70, 1253-1261. 4) T. Kida et al., Tetrahedron 2005, 61, 5763-5768. 5) A. Kikuzawa et al., Org. Lett. 2007, 9, 3909-3912. 6) A. Kikuzawa et al., Macromolecules 2008, 41, 3393-3395.
5.Chiral Photochemistry through the Charge-Transfer Band Excitation
Upon photoexcitation, molecules are excited in their electronically excited states and gain additional reactivity. Accordingly, electronically excited molecules readily undergo unique photochemical transformation that does not usually observed in the ground state. Electron donor and acceptor molecules interact each other in their ground state to form charge-transfer complex, in which new absorption band (i.e., CT band) is observed. Upon selective excitation at the CT band, the complex is excited to form excited CT complex. This excited species behaves rather differently than the conventional exciplex, generally formed in the direct photoreaction. We are perusing the new methodology for chiral photoreactions based on the selective CT excitation.
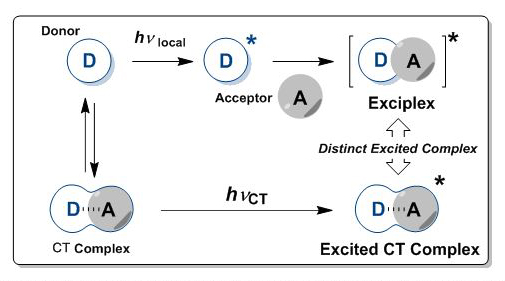
Figure 6. Chiral Photoreactions through the Selective Charge-Transfer Band Excitation (Top: Conceptual View, Bottom: Representative Example of Photoreaction).
- 1) T. Mori, Y. Inoue, Chem. Soc. Rev. 2013, 42, 8142. 2) T. Mori, Y. Inoue, Photochemistry in Alternative Media in CRC Handbook of Organic Photochemistry and Photobiology (3rd Ed.) 2012. 3) T. Mori, Y. Inoue, Top. Curr. Chem. 2011, 298, 99. 4) T. Mori, C. Bohne, Photochem. Photobiol. Sci. 2011, 10, 13
6.Development and application of highly luminescent circularly polarized emitting molecules with molecular recognition properties
In this study, we have successfully developed molecules that emit circularly polarized luminescence (CPL), a unique type of light that rotates while propagating, by employing a novel strategy of modifying cyclodextrins with numerous luminescent groups. Through the modification of various luminescent groups, we have demonstrated that cyclodextrins can transform various dyes into highly luminescent CPL molecules. Furthermore, we aim to achieve novel CPL biosensing and imaging utilizing the molecular recognition ability and chirality of cyclodextrins, thereby contributing to the field of biology.
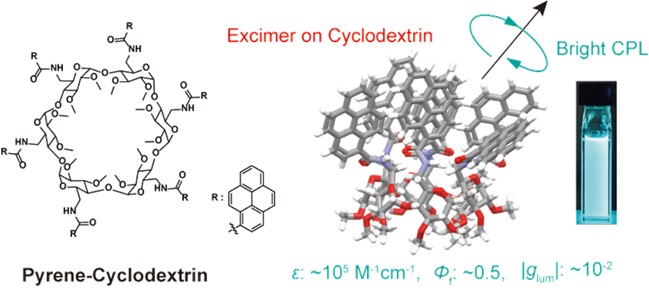
Figure 7. Bright CPL molecules composed of a cyclodextrin.
- 1) H. Shigemitsu et al., Angew. Chem. Int. Ed. 2022, 61, e202114700. 2) H. Shigemitsu et al., Asian J. Org. Chem., 2020, 9, 2112-2115.
7.Creation and utilization of supramolecular photocatalysts through self-assembly of organic dye molecules
In our research, we are exploring the development of photocatalysts based on "supramolecular assemblies" formed by the self-assembly of organic molecules. We have discovered that rhodamine, a well-known organic dye molecule, acquires photocatalytic activity through self-assembly in water. Previously, rhodamine has been primarily recognized for its excellent luminescent properties, and its aggregated state, which leads to the loss of these properties, was considered undesirable. However, it has become apparent that this aggregated state not only diminishes luminescent characteristics but also confers photocatalytic activity. This principle has been found to be applicable to various organic dye molecules, and we are advancing research on photocatalysts based on molecular assemblies, referred to as "supramolecular photocatalysts."
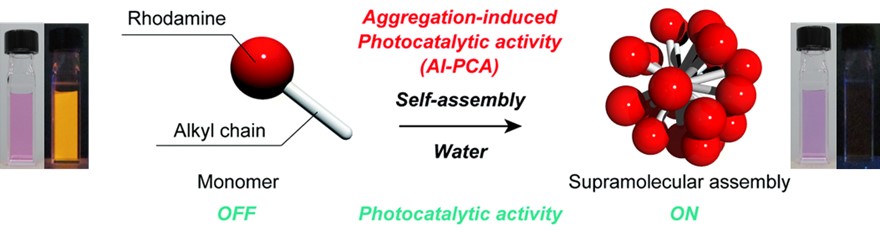
Figure 8. Self-assembly induced photocatalytic activity and supramolecular photocatalysts.
- 1) A. Bunno et al., Chem. Commun., 2024, 60, 889–892. 2) H. Shigemitsu et al., JACS Au, 2022, 2, 1472–1478. 3) H. Shigemitsu et al., ACS Appl. Nano Mater., 2022, 5, 14954–14960. 4) H. Shigemitsu et al., Chem. Commun., 2021, 57, 11217–11220. 5) H. Shigemitsu et al., Chem. Sci., 2020, 11, 11843–11848.
- Home
- Research